Piezoelectric Materials – The Most Common Unknown Power Source
With new practical applications being developed every day, the piezoelectric industry is expected to reach roughly 41 billion within the coming three years, with a compound annual growth rate of nearly 6%. This boom will allow for the further development and implementation of high-tech amorphous and film-based piezoelectric polymers in the modern world.
What are Piezoelectric Materials?
Piezoelectric materials allow for us to harness kinetic energy, by transforming force into an electric charge. First defined by the Curie brothers in 1880, Piezoelectricity has become a fundamental principle exploited in modern technology.
Piezoelectricity refers to a substances ability to produce an electrical charge when mechanical stress is applied. This electrical charge is produced by forced asymmetry. In piezoelectric materials, positive and negative charges are separated from each other, while remaining aligned in a symmetrical pattern. When mechanical stress is applied to the substance, this symmetry is lost, resulting in the production of an electric charge.
Another unique property of the materials is the random nature and presence of Weiss domains (magnetically oriented without external magnetic influence).
It was later discovered that these same materials demonstrated a direct inverse property to the electric effect. It was found that if an electric charge was applied to the material, repeatable mechanical deformation would occur within the material. This discovery gave great utility to such materials, as it essentially doubled their prospective use-cases.
Manufacturers and Innovators
Before we dive in to examples of real-world use-cases, the following are a trio of leading companies that leverage piezoelectric materials throughout a variety of products integral in modern electronics.
Notably, analysts for Barron’s currently list each of the following stocks as either ‘over’ or ‘buy’.
Stoneridge (SRI)
Listed on the NYSE, Stoneridge (SRI) has seen its shares increase in value over the past year by more than 30% at time of writing. While revenue at Stoneridge took a hit during the height of COVID, 2021 saw a nearly 20% rebound to 770M
Stoneridge employs over 5,000 people, and operates out of the State of Michigan.
Methode Electronics (MEI)
Listed on the NYSE, Methode Electronics Inc. has seen its shares increase in value over the past year by nearly 15% at time of writing. Over the past 4 years, Methode Electronics has managed to continue growing its revenue between 2.36% and 10.13% each year. For 2022, revenue topped 1.16B.
Methode Electronics employs over 7,000 people, and operates out of the State of Illinois.
Kimball Electronics Inc. (KE)
Listed on Nasdaq, Kimball Electronics Inc. has seen its shares increase in value over the past year by more than 32% at time of writing. Where the companies listed above struggled from 2019-2020, Kimball Electronics managed to continually boast increasing revenues. Totaling 1.35B for 2022, this marks a 4.47% increase over 2021.
Kimball Electronics employs over 7,000 people, and operates out of the State of Indiana.
Modern Advancements
Traditionally, naturally occurring piezoelectric substances were used to demonstrate the effect. Most commonly, the material of choice was quartz. When the limits of naturally occurring substances were reached, man-made ceramics became the popular choice. Designed in 1952, and still one of the most popular piezoelectric ceramic today is PZT (lead zirconate titanate). However, with drawbacks such as limited deformation, fragility, and a high mass density, PZT is not ideal for every application.
In 1964 PVDF (poly vinylidene fluoride) was developed. PVDF has a semi-crystalline structure and creates charges several times greater than quartz. Although this man made polymer addressed many of the drawbacks of PZT, it had various of its own – piezoelectric breakdowns at high temperatures, and degradation. With recent technological advancement and increasing demands, PZT and PVDF may have reached their limits.
In the early 2000’s institutes such as GAIKER-IK4 began to develop what are known as amorphous piezoelectric polymers. By utilizing an amorphous structure, much higher temperatures can be endured by the substance. Since the piezoelectric effects are not relying on the crystalline structure which breaks down at higher temperatures, the amorphous structures make for a much more rugged polymer.
These amorphous polymers are being developed because they offer higher levels of deformation, large weight reduction, and greater ruggedness. By achieving this, the field of applications for the materials now allows for the incorporation of aerospace and electronic devices. With the new amorphous piezoelectric polymers and films being developed, failure during use will occur at temperatures of roughly 150°C and greater. Degradation of the substance will occur at roughly 400°C. While this may limit their use in extreme conditions, the vast majority of applications fall within an appropriate range.
Like many new substances, these polymers are being developed by using PVDF and PVT as the fundamentals. Attempting to keep positive attributes from each material while eliminating as many disadvantages as possible. Although such products are newer polymers, they are modeled after the current working models.
By utilizing an amorphous structure, extensive testing must be done on optimum vitreous transition temperatures. This value is directly linked to the strength of piezoelectric properties the material will possess. The amorphous structure demonstrates and relies on short range order to produce a piezoelectric effect instead, of long range order as seen in crystalline structures. In addition to this, many are opting to incorporate polyimides into the structure of the materials due to their mechanical, dielectric, and thermal properties, with the polyimides ensuring poling of molecules regardless on their positioning.
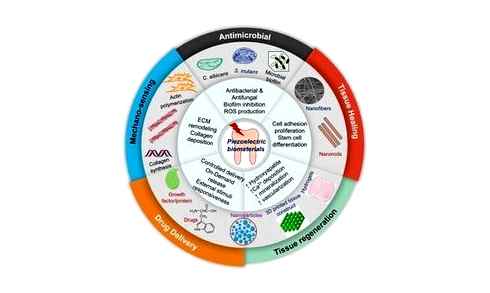
Use-Cases
Past and current applications of piezoelectric materials include many inconspicuous items such as lighters, quartz clocks and even engine management systems. The most common use for them currently would be in sensors and actuators. While suitable piezoelectric materials have been applied for these use cases, future applications demand a more versatile material. Thankfully developing piezoelectric polymers are just that – versatile. With constant advancements in our understanding of material science, and their ability to display direct inverse effects, the number of applications in which they can be used continues to increase. Some intriguing present and potential future applications include,
Mobile and Wearable Electronics
Talk powered cell phones and wearable devices. By utilizing the pressure created within the microphone due to sound waves, piezoelectric polymers can hopefully one day create enough power needed to use the phone. While it is unlikely that this concept will remove the need for a battery altogether any time soon, it does create the possibility of extending battery life in low-drain wearable Smart devices.
It should be noted that piezoelectric materials have been used in microphones for nearly 100 years at this point. Rather than the end goal being to charge a device though, these applications allow for the conversion of soundwaves in to electricity for the purpose of recording and playback in a cost-effective manner.
Dampening Systems
Another application is the use of piezoelectric materials in dampening systems. Companies such as HEAD have incorporated this idea into its tennis rackets and skis in an effort to absorb/dampen vibrations. When an impact occurs on the racket or ski, the reciprocal effect is harnessed by sending the electric signal created to an inverse material providing an opposing force. This results in an effective dampening system.
This same concept is being applied to noise and vibration reduction in cars, homes, and in hazardous workplace environments. One example of such an environment would be Bitcoin mining farms. Not only are vibrations harmful to electronic equipment over the long run, there have been various instances of surrounding communities in which these operations take place complaining about the resulting noise and vibrations resulting from the use of ASIC devices. In many similar scenarios, piezo-based actuators are used as a solution to dampen each of these effects. With sound waves being created in cars, homes, and machinery by materials reverberating, this noise can also be eliminated, or at least reduced, with traditional methods such as an adhesive dampening material. These materials work passively though, and are very heavy and expensive. They typically work by lowering a materials resonant frequency. Exploiting the properties of piezoelectric polymers solves this problem by taking the more active and dynamic approach described above.
Cleaning Solutions
To demonstrate just how versatile the use cases for piezoelectric materials are, consider work being done by companies like Solar PiezoClean. In this instance, the company is coating solar panels with a piezoelectric film. The purpose is to offer a low maintenance means of keeping solar panels clean – a key to ensuring optimal efficiency.
This process involves applying an electric charge to the film, which then vibrates at a specific frequency and pitch that allows for dust and dirt to simply fall off with help from gravity. What this all means is savings in water and manpower, while increasing longevity and efficiency of coated panels. A simple, but ingenious solution to a problem that is only growing as solar installations become more commonplace.
common implementations of piezoelectric materials in such a manner include ultrasonic cleaning devices like jewelry cleaners.
Aerospace
Earlier we mentioned the use of piezoelectric materials within the aerospace sector. Here, planes can make use of such materials to monitor structural integrity and stressors through the measurement of electric charges produced – a use case that can allow not only for increased safety, but greater efficiencies by allowing for engineers to simultaneously cut weight and strengthen structures where needed.
Move beyond our atmosphere, and piezoelectric actuators are used in many satellites. The ability to operate with extreme precision allows for such actuators to make micro-thrusters capable of proper satellite positioning.
HealthCare Diagnostic Tools
As our ability to create smaller and smaller devices improves, we are now using piezoelectric materials in various diagnostic tools within healthcare. An example of this is Intravascular Ultrasound (IVUS). IVUS is a process which allows for tiny probes to generate imaging from within blood vessels. This is done through the use of ultrasound transducers built with piezoelectric single crystals.
Piezoelectric materials are also used in certain dentistry equipment. Similar to the cleaning solution being utilized by SolarClean described above, this equipment relies on ultrasonic waves, produced by applying an electrical current to the piezoelectric materials, to clean/remove plaque from teeth.
Sonar
Sonar (Sound Navigation and Ranging) systems can be used to provide imaging, or for communication. Examples of imaging include topographic mapping of ocean floors, or every-day fishfinders. Meanwhile communication can be achieved through the creation of sound waves. Each of these processes are made possible through the use of piezoelectric transducers.
Despite being developed over 100 years ago, Sonar continues to play an important role today. The most recent widespread example of this would be its implementation in self-driving cars, which typically use a combination of Sonar, LIDAR, and radar to track and interpret surroundings.
Energy Harvesting
Finally, a very intriguing application would be large scale energy production. Piezoelectric polymers are being developed to place in high traffic areas, including various factories, sport fields, train stations, and more around the world. A 1cm 3 piece of quartz is capable of producing up to 4,500V of electricity when 175lbs of force is applied. With each footstep to hit the ground in such stations creating this electricity, there is the potential to harness huge amounts as it is created daily – greatly increasing efficiency and electricity costs for the building.
Beyond foot traffic, many have envisioned a future in which roadways are embedded with such materials, creating electricity to power street lights and signs as cars exert physical force on them.
When combined, future technologies like wireless car charging being developed by Electreon, and powered surfaces by companies like Pavegen, will hopefully one day allow for reduced battery sizes in vehicles, and a much more efficient and clean way to keep electric vehicles charged.
Final Word
Overall, the potential of piezoelectric materials is just beginning to be realized. Photovoltaic effects that make Solar energy possible were discovered in the mid-1800’s, and are only now becoming practical for widespread use. Piezoelectric materials are no different, and as research and development in to these materials continues, increases in efficiency and durability follow suit. Modern scientific advancements are only now allowing us to realize, or at least understand, the full potential of this source for energy, with the use-cases listed here (electricity generation, sound dampening, sonar, sensors, acutators, etc) being only a select few out of countless possibilities.
Piezoelectricity Is the Renewable Energy We’ve Been Waiting For
First discovered by Pierre Curie, the husband of Marie Curie, and his older brother Jacques in 1880, piezoelectricity works by taking an electrically neutral substance such as particular crystals, ceramics, and even biological materials and applying enough pressure to create an imbalance of positively and negatively charged atoms on opposite sides. Under normal conditions, the arrangement of the atoms balances out the electric charge, but under pressure, an electric field can be created because the neutral arrangement has been disfigured, leaving a higher concentration of positively charged atoms on one side and negatively charged atoms on the other.
The piezoelectric effect has a variety of applications today, such as in quartz watches, BBQ lighters, guitar pickups, clocks in electronics, inkjet printers, etc. The reason you have to push the button so hard on BBQ lighters, for example, is because you’re actually slightly deforming a quartz crystal, which creates an electric charge that is then forced to jump a tiny gap, creating a spark and igniting the lighter fluid.
So if any sort of movement has the potential to become source of electricity, why are we not using this more often? In the last few years, researchers have been working on implementing piezoelectric materials into the human body, roadways, bike paths, sidewalks, solar panels, etc. to unlock this relatively untapped source of renewable energy.
Shock to the Heart
In a paper published in Nature, Professor Ehud Gazit and his team from Tel Aviv University demonstrated their use of the piezoelectric effect to convert both voluntary and involuntary body movements to an internal electric current strong enough to power medical devices. Everything from bowel movements to the expansion and contraction of the lungs is a potential source of energy. They claimed that “The piezoelectric effect in proteins is an intriguing phenomenon that can potentially allow a better interface between the semiconductor and biological worlds.”
This idea isn’t new, but its uses were limited because researchers couldn’t find materials that were both safe and electrically efficient. The most common piezoelectric material used commercially is lead zirconate titanate, a type of ceramic, which of course contains lead, making it toxic. However, Gazit et al. engineered a nanomaterial material that mimics collagen, the most prevalent protein in the body. Through their clever use of self-arranging peptides, their new material produced an electric current that rivals or possibly exceeds commercial materials. They said “we fabricated a simple biopiezoelectric device made from collagen-mimicking ultra-short peptide sequences that could achieve high current and voltage output, similar to that obtained using nanogenerators comprising inorganic materials or organic polymers.”
Therefore, thanks to piezoelectricity, new medical devices will have their own safe power supply, allowing for longer-lasting and more advanced pacemakers, defibrillators, blood sensors, drug delivery systems, and whatever else is coming with next-generation medical devices.
On the Road Again
Given the amount of cars and buses on the world’s highways and the amount of people using sidewalks and bike paths, it’s safe to say that a staggering amount of energy could be collected through piezoelectricity. Every time a car exerts a force on the roadway, a pedestrian steps on the sidewalk, or a cyclist rolls down a bike path, this mechanical energy could be converted to electricity. Fortunately, researchers are working on it.
For example, a research team from Lancaster University is testing a variety of piezoelectric materials and configurations, and the initial results look promising. They found that under normal traffic conditions (2000 to 3000 cars per hour) they can generate around 2 MW per kilometer of road, enough to power 2000 to 4000 street lamps. Taking into consideration the cost of installing this new energy collecting technology, the researchers believe they could save the city 20% of its cost to electrify their roadways. Lead researcher Professor Saafi said “The system we develop will then convert this mechanical energy into electric energy to power things such as street lamps, traffic lights and electric car charging points. It could also be used to provide other Smart street benefits, such as real-time traffic volume monitoring.”
Similarly, California has invested 2.3 million on two projects to test the viability of harvesting energy from the mass movement of people. One of these projects is a 60 meter long stretch of road near the University of California, Merced. This roadway will be peppered with 2 centimeter wide stacks of piezoelectric ceramics. The other project is run by Pyro-E, a San Jose based LLC, and with a similar strategy they believe they can generate enough electricity to power 5000 homes. If scaled up, they believe “the power generated could provide 60% rate-reduction from retail electricity to help offset the adverse environmental impact of gasoline vehicles.”
Furthermore, researchers have been pushing forward with paving roads with solar panels. If/when this happens, it turns out that piezoelectricity can make them more efficient. In a paper published by the Royal Society of Chemistry, the authors demonstrated that solar cells made with CH3NH3PbI3 change their efficiency when compressed. They claim that “While an external strain is applied in the CH3NH3PbI3 layer, the performance of the PPSC [piezo-phototronic organic perovskite] improves linearly.” Therefore, a roadway paved with solar cells would be great, but a roadway paved with piezo-solar cells would be much more efficient, with the more strain the better.
Of course, all of this adds to the cost of maintenance and installation, but researchers are confident that new materials and configurations can turn enough of a profit to make it worth while.
Blowing In the Wind
Harnessing the wind is best done through air turbines, but even with the best designs energy is still left on the table. All structures exhibit As air flows around a structure it can become turbulent due to a process known as vortex-induced vibrations (VIV), in which air is forced to bend according to the curvature, in some cases creating a vortex on the structure’s surface. When this doesn’t happen symmetrically, the structure vibrates. When the vibrations match the structure’s resonant frequency, the structure oscillates heavily. Likewise, the galloping wind effect can be another source of energy. As strong wind pushes into and a around a structure, it exerts forces that compete with the structure’s natural elasticity, causing large oscillations.
Researchers believe energy from both VIV and the galloping wind effect can be tapped. A team of researchers from China demonstrated in a paper from the Journal of Sensors that a “square cylinder” is an ideal shape for generating piezoelectricity from the wind. As this shape is hit by the wind, it oscillates, compressing piezoelectric ceramics at its base, producing a current. The authors said that “It is worth noting that both vortex-induced vibration and galloping of a square-section structure can be utilized in the same piezoelectric wind energy harvesting equipment, because both of the two phenomena can cause large oscillating amplitude that can be good power source to excite the piezoelectric device to vibrate.” They envision implementing their idea into already existing wind energy devices, adding to their efficiency by harnessing energy that would’ve otherwise gone unused.
Is Piezoelectricity Worth It?
Piezoelectricity has faced an uphill battle with large-scale implementation. For one, piezoelectric materials are sensitive to high temperatures, in that they become less efficient when subjected to heat. Second, piezoelectric crystals are water soluble, meaning they must be protected against the elements, creating additional costs. Third, and most importantly, piezoelectricity doesn’t produce enough output to compete with other forms of energy creation.
However, the field of piezoelectricity is pushing forward quickly, creating better materials and configurations. For example, the US Navy just invested a few million dollars to develop “next generation piezoelectric single crystal materials” which is likely to result in piezoelectric materials with far greater output and durability. Because of research like this and the others discussed above, in only the last few years, we’ve seen numerous advancements that’ve made this form of energy creation increasingly more attractive. So membranes that can power internal medical devices, roadways that light themselves, and more efficient wind turbines, are just a few examples on a growing list of applications.
Support The Happy Neuron by clicking the links below:
Piezoelectricity: using citizens’ footsteps to generate electricity
Piezoelectricity, together with photovoltaics, is seen as one of the options with the most potential in the generation of urban energy. Distributed, clean, it uses the mechanical energy of citizens when walking to power all types of urban elements, from lighting to edge computing devices or IoT. With energy consumption on the rise, this is another way of covering the demand of low consumption electronic components.
What is piezoelectricity and what does it consist of?
Piezoelectricity is a physical phenomenon via which mechanically stressed crystals generate potential differences. Quartz is one of these piezoelectric crystals. By squeezing and stretching it, it generates a slight electrical potential difference between its ends by rearranging its charges. If a cable is connected between them, a usable micro-current can be formed.
Who discovered piezoelectricity?
The fact that piezoelectricity is still a somewhat unexplored phenomenon could lend to believe that it’s a modern discovery, and nothing could be further from the truth. Piezoelectricty was theorized close to two centuries ago by René Just Haüy and Antoine César Becquerel after investigating the pyroelectric effect, by which a material generates an electric potential in response to a temperature change.
Nevertheless, the first demonstration of piezoelectricity wouldn’t happen until 1880, when brothers Pierre Curie and Jacques Curie discovered that applying pressure to crystals such as quartz, topaz, tourmaline and even cane sugar generated electrical charges on their surface.
For years, piezoelectricty would remain a curiosity, until World War I unraveled, and the war effort pushed diverse technologies to their limits. The development of sonar devices, built from quartz crystals, was among the first practical uses for it.
How does piezoelectricity work?
As with any other form of electrical current, the energy generated through piezoelectricity can be used, but its quantity is somewhat limited; it is around 20 watts (in comparison, a mobile phone charges at 15 watts). To use this electricity, electric generators need to be installed in busy areas, such as in the reception area of buildings, a subway platform or the weight machine area of a gymnasium.
To avoid losses through significant distances, the energy channeled with the system is consumed very close to the point at which it is generated. Often within just a few meters. For example, it can power nearby telephone devices.
Is this technology efficient since it generates so little?
The truth is piezoelectric systems are quite robust and sufficiently basic to ensure high durability. Furthermore, the energy cost of manufacturing these panels, compared with more common elements, such as ceramics, (11.1 MJ/kg). is not high.
Imagine if one of these panels has a conventional concrete cover (1.3 MJ/kg) and it weights around 5 kg. Those 6.5 MJ (6,500,000 J) seem like a lot, but even with a generation of 10 W (10 J/s, it would only take 650,000 seconds to amortize the energy investment. That is 180 hours of steps, which is ridiculously low compared with the years or decades that they can last.
Yes, this calculation would have to include mechanical and metal parts, perhaps doubling or tripling those amortization hours. But they are still far below the useful life of the generator. It is a Smart urban option.
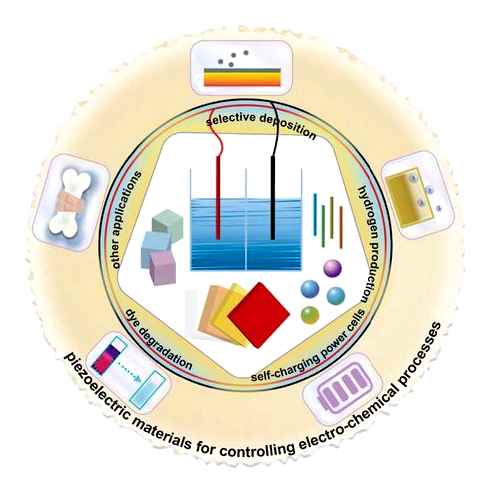
What could piezoelectric energy be used for?
It is important to think of low-consumption applications. Apart from being used to ignite lighters, piezoelectricity is already being used in pilot projects in which the pavement generates sufficient energy to keep the lights switched on (Pavegen).
Other brands, such as Israel’s Innowattech and the Californian Pyro-e, extract energy form the movement of vehicles on the road. The system is the same, but it is the car that generates energy.
The role of piezoelectricity in the sensorization of Smart cities
A rural environment with a low population does not seem like the ideal place for piezoelectricity (in this case a solar floor is more appropriate), unlike densely populated cities.
It is precisely in cities where big data that relates the movement of citizens with its services is particularly relevant, where most data are consumed or where there are edge computing services. These services, as with operators’ virtualized networks ‘bring’ internet services to users, consuming closer to them and less on servers on the other side of the world. And they need to be given energy.
Thin-film flexible piezoelectric-photovoltaic cell based on selenium
Japanese scientists have developed a piezoelectric-photovoltaic cell with potential applications in indoor environments. They fabricated it on a flexible polyethylene terephthalate film with sputter-deposited ZnMgO thin film and photo-absorbing selenium as the n-type window layer and p-type layer, respectively.
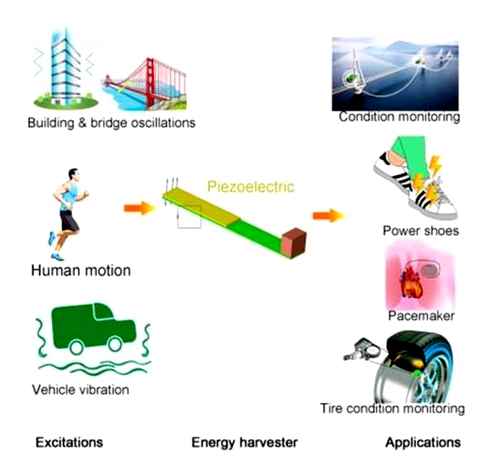
The ZnMgO/Se heterojunction device has an open-circuit voltage of 0.75 V.
Image: Ritsumeikan University,
Share
Researchers from Ritsumeikan University in Japan have built a heterojunction solar cell based on zinc magnesium oxide (ZnMgO) and selenium (Se).
It works through the so-called piezoelectric effect. Piezoelectric materials are commonly used for engineering applications, mainly as actuators and sensors generating and sensing mechanical deformation in materials.
“ The effect of piezoelectric materials polarized by mechanical strain or vibration on photovoltaic or photocatalytic performances is called the piezo-phototronic effect,” the scientists said. “The piezo-phototronic effect has attracted attention for improving the performances of photovoltaic devices and LEDs by rearranging the energy bands at the p-n junction.”
Researcher Taizo Kobayashi said the cells could be produced at lower costs than copper, indium, gallium and selenium (CIGS) or perovskite thin-film solar cells, as the p-type selenium used in the devices is an inexpensive element.
“Potential applications are thin-film flexible piezoelectric-photovoltaic devices that can generate electricity simply from indoor lighting produced by LEDs and compact fluorescent lamps, or self-powered strain sensing devices,” Kobayashi told pv magazine.
The group fabricated the solar cell on a flexible polyethylene terephthalate (PET) film with sputter-deposited ZnMgO thin film and photo-absorbing selenium as the n-type window layer and p-type layer, respectively.
“When compressive strain is applied on the ZnMgO layer, which is piezoelectric, wurtzite and semiconducting, a negatively polarized ZnMgO region is formed near the Se layer,” the scientists said.
Popular content
The polarization decreases the c onduction Band offset ( CBO) at the interface of ZnMgO and Se layer. The CBO is the energy difference between bands of materials when considering a semiconductor heterostructure.
“ZnO has a non-centrosymmetric wurtzite-type crystallographic structure which can induce piezoelectric polarization charges on its surface under strain,” said the scientists.
Wurtzite zinc oxide (ZnO) is a remarkable multifunctional zinc sulfide mineral with a hexagonal structure, a distinctive property set, and a huge range of existing and emerging applications. The ZnMgO piezoelectric layer is deformed to negatively polarize ZnMgO/Se interface. As a result, the positive piezoelectric polarization charges can be generated at the interface between the ZnMgO and indium-tin-oxide (ITO) layers. This is turn forms a barrier that facilitates carrier collection from both layers via excessive negative polarization.
The scientists applied different strain levels to the ZnMgO/Se interface and the cell performance was measured to assess the impact of this variation. When the applied strain was increased from 0.41% to 0.40% by varying the bending angle, the device’s open-circuit voltage increased from 0.59 V to 0.75 V. The scientists claim this paves the way for significant efficiency improvements for ZnMgO/Se heterojunction photovoltaic devices based on piezo-phototronic effects.
They introduced the new cell concept in “ Impact of piezo-phototronic effect on ZnMgO/Se heterojunction photovoltaic devices,” which was recently published in Nano Energy.
“Since ZnMgO/Se heterojunction can be fabricated by only common sputtering and evaporation system, it is considered that it is not difficult to fabricate the proposed device commercially,” said Kobayashi.
This content is protected by copyright and may not be reused. If you want to cooperate with us and would like to reuse some of our content, please contact: editors@pv-magazine.com.
Emiliano Bellini
Emiliano joined pv magazine in March 2017. He has been reporting on solar and renewable energy since 2009.